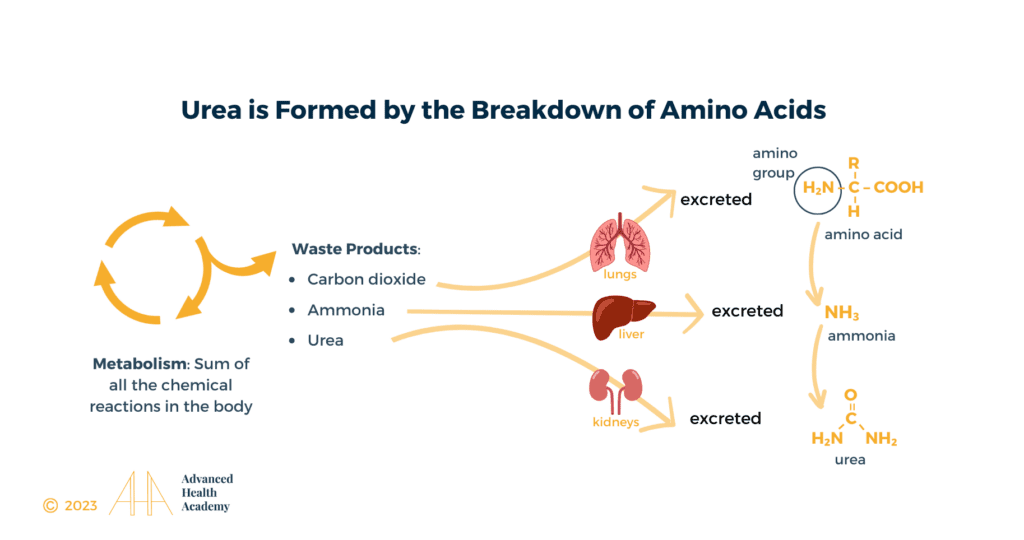
What is BUN?
One of the blood markers the Complete Metabolic Panel (CMP) looks at is blood urea nitrogen (BUN). BUN is formed in the liver during the final step of protein metabolism. Urea is a waste product that your kidneys remove from the blood. The blood urea nitrogen (BUN) test measures the amount of toxic protein waste products in the blood that the body has not excreted. It is also referred to as the “BUN, serum” or ”BUN, plasma” test.
What is nitrogen, and why is it important?
All life on Earth requires nitrogen for many processes. Most fundamentally, life requires nitrogen as it is an essential part of amino acids and nucleic acids.
The air we breathe is 80% nitrogen gas (N2) and 20% oxygen. Plants and photosynthetic bacteria in nature are the primary producers of nitrogen. Plants cannot utilize nitrogen gas, but bacteria can. On land, photosynthetic bacteria live in the soil and are attached to plant roots. In water, these bacteria float freely.
Particular bacteria contain the enzyme nitrogenase, which is critical for converting nitrogen gas (N2) to ammonia (NH3) or ammonium (NH4). Through oxidation, certain bacteria turn ammonium into nitrite (NO2), while others convert nitrite (NO2) into nitrate (NO3).
Nitrate (NO3) is a more stable molecule than nitrite (NO2). It is the best source of nitrogen for primary producers. The roots transport nitrate to the chlorophyll in plant cells to convert sunlight into energy (called adenosine triphosphate; ATP).
If nitrogen is limited, which it often is, chlorophyll is also limited. Limited chlorophyll means limited photosynthesis. Limited phosphate means less ATP production. In other words, there is less overall energy in the food web for all life on Earth to benefit from.
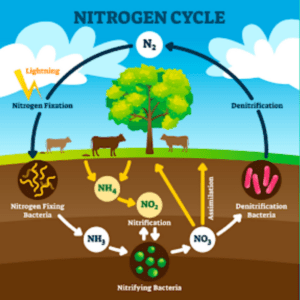
Nitrates (NO3) are naturally found in plants rich in chlorophyll, such as dark leafy vegetables (e.g., kale, arugula, swiss chard, spinach), beets, cabbage, cauliflower, carrots, and broccoli. Nitrates are not naturally found in meat, fish, and dairy products but are added by processing plants for preservation.
When we chew food, healthy bacteria in the mouth react with saliva and break food down into small fragments. Proteins are broken down into amino acids, and nitrates are converted into nitrites. Once it passes through the esophagus to the stomach, hydrochloric acid and digestive enzymes (e.g., pepsin) are released to break food further and turned into a uniform mixture called chyme. Nitrites are converted to nitric oxide (NO), which goes into the bloodstream to meet the body’s needs.
Nitric oxide acts as a messenger to relax the smooth muscles within the lining of the arteries. When the muscle relaxes, the arteries widen, allowing for increased blood flow and circulation. Nitric oxide keeps arteries young and flexible, prevents plaque from building up on vessel walls, keeps blood pressure under control, and reduces the formation of blood clots. Nitric oxide protects the body against pathogenic microorganism and cancer cell overgrowth and helps brain cells communicate and reduce the risk of dementia.
Leftover nitrates and nitrites that are not converted to nitric oxide in the stomach are absorbed entirely in the small intestine. 60 percent of nitrates and nitrites are excreted in the kidneys. The rest are transported back to the mouth and stored in the salivary glands or under the skin. Bacteria in the mouth and on the skin are responsible for the converting nitrates and nitrites into nitric oxide.
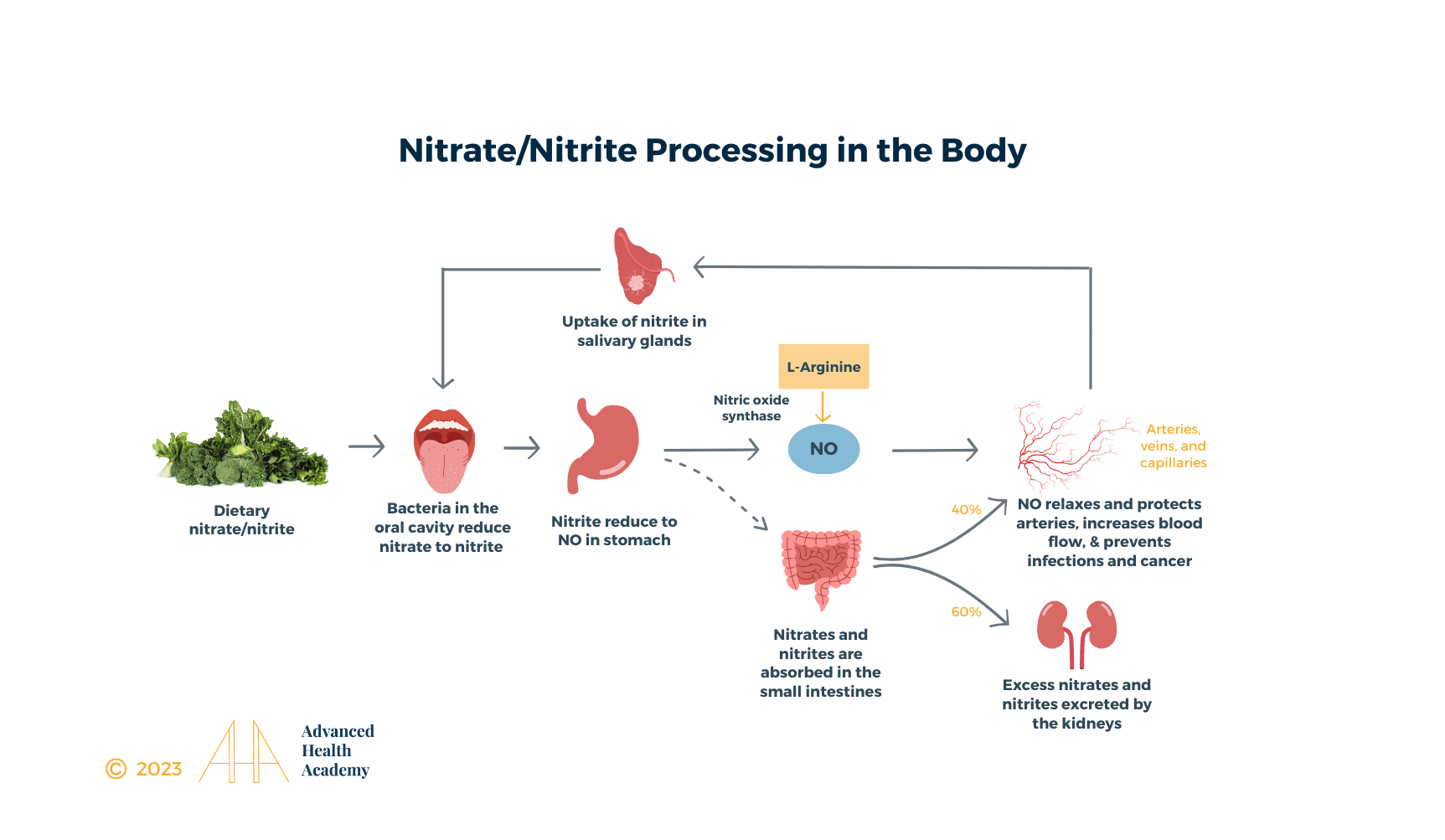
What is urea and why is it important?
Urea is a small organic molecule comprising two amino (NH2) groups and a linked carbamyl (C-O) group:
Urea is the principal nitrogenous end-product of protein metabolism.
Much of the body is made of protein, and these proteins take on a myriad of forms and functions. They represent cell signaling receptors, signaling molecules, structural members, enzymes, intracellular trafficking components, extracellular matrix scaffolds, ion pumps, ion channels, oxygen and CO transporters (hemoglobin). Protein is in bones (collagen), muscles (creatine), and tendons; the hemoglobin that transports oxygen; the enzymes that catalyze all biochemical reactions; and those responsible for growth and repair. Amid all these necessary functions, proteins also serve as an energy source.
Every day, over 250 grams of protein in your body are dismantled, and 250 grams of new protein are built. Proteins are long chains of amino acids that are connected to each other by peptide bonds. An amino acid contains an amino (-NH2) and carboxylic acid (-COOH) functional group, hence the amino acid. Proteins are unique based on which amino acids they contain, how they are arranged in a chain, and the complex interactions the chain makes with itself and the environment [20][21].
Protein Metabolism
The digestion of protein derived from food begins in the stomach. The enzyme pepsin and hydrochloric acid (HCl; 0.5 percent) produces a pH of 1.5–3.5 that denatures proteins into smaller polypeptides and their constituent amino acids. This food-gastric juice mixture (chyme) then enters the small intestine, where the bacteria plays the most significant role in releasing enzymes, including secretin and cholecystokinin (CCK), critical for breaking apart proteins into individual amino acids. The bacteria also metabolize vitamins and ferment indigestible carbohydrates (plant-derived dietary fiber) to short-chain fatty acids (e.g., acetate, propionate, butyrate). Secretin stimulates the pancreas to release sodium bicarbonate and most of the digestive enzymes, including the proteases trypsin, chymotrypsin, and elastase, which aid protein digestion and neutralize the HCl (essential for protecting the intestines’ lining). Individual amino acids are then transported across the small intestines, mediated by sodium-amino acid transporters, and into the bloodstream to be transported to the liver and cells throughout the body.
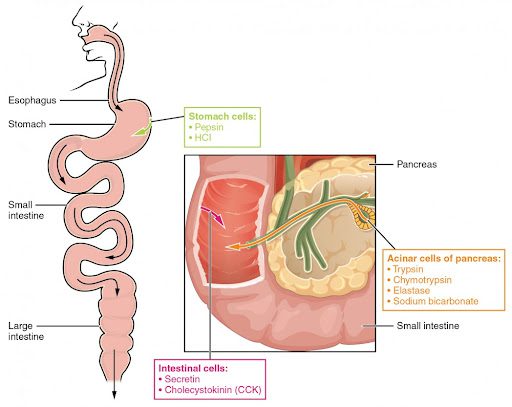
Urea Cycle
The processing of amino acids results in the creation of metabolic intermediates, including pyruvate, acetyl CoA, acetoacetyl CoA, oxaloacetate, and α-ketoglutarate, which are used as energy sources via the Krebs cycle; hydrocarbons, and toxic toxic amino groups (-NH2). NH2 is degraded in the liver to ammonia (NH3) and then converted to less toxic urea, carbon dioxide (CO2), and water. Urea is released into the bloodstream and transported to the kidneys, with 40-50% appearing in urine. Sweat, stool, and exhalation eliminate less than 10% of urea. The remaining 60-70% of urea is stored as fat in the liver, abdominal cavity, and muscle tissue or accumulates in the blood.
Why Does BUN Matter
The diet’s protein content influences the urea production rate; a low-animal protein diet is associated with reduced urea production, and a high-animal protein diet is associated with increased urea production. Malnutrition is also associated with increased urea production caused by the increased metabolism of muscle protein used as an energy source. Any pathology associated with tissue breakdown is, for the same reason, associated with increased urea production[8].
Normal Range
In the US and a few other countries, plasma or serum urea concentration is expressed as the nitrogen content of urea (molecular weight 28) and reported as blood urea nitrogen (BUN) in non-Standard International (SI) units (mg/dL). In all other parts of the world, urea results are expressed as the whole urea molecule (not just the nitrogen part of the molecule; molecular weight 60) and reported as urea in SI units (mmol/L). Since BUN reflects only the nitrogen content of urea (MW 28) and urea measurement demonstrates the whole of the molecule (MW 60), urea is approximately twice (60/28 = 2.14) that of BUN[9].
Thus, BUN 10 mg/dL is equivalent to urea 21.4 mg/dL.
To convert BUN (mg/dL) to urea (mmol/L):
Multiply by 10 to convert from /dL to /L and divide by 28 to convert from mg BUN to mmol urea, i.e. 10/28 = 0.357
So the conversion factor is 0.357
BUN mg/dL multiplied by 0.357 = urea (mmol/L)
Urea (mmol/L) divided by 0.357 = BUN (mg/dL)
It is widely accepted that there is an age-related increase in plasma/serum urea concentration [9],[10], but this needs to be better defined. There is uncertainty as to whether it simply reflects an age-related decline in renal function, as some studies [11] suggest, or occurs despite normal renal function, as others [12] seem to suggest. The results of [12] indicate that healthy elderly individuals (without any apparent loss of renal function) may have BUN levels as high as 40-50 mg/dL (14.3-17.8 mmol/L)].
The normal range of BUN is considered 6 to 25 mg/dl, or 2.7 to 7.8 mmol urea per liter (SI units).[10] Laboratory Minimum and Maximum is increased by 1 mg/dL for adults > 60 years.[2]
Optimal Range
The most up-to-date medical research shows that the optimal range of blood urea nitrogen is one standard deviation from lab mean or 4.1 to 18 mg/dl, or 1.46 to 6.4 mmol urea per liter.
Warning Range
The most up-to-date medical research shows that organs are not functioning correctly when the BUN is 18 to 25 mg/dl, or 6.4 to 8.9 mmol urea per liter. The range is wide because of normal variations in protein intake, protein catabolism, and how efficiently protein’s toxic byproducts are excreted from the body.
High BUN Levels (i.e. urea >8.9 mmol/L or BUN >18 mg/dL)
Serum/plasma urea concentration reflects the balance between urea production in the liver and urea elimination by the kidneys, in urine; increased plasma/serum urea can be caused by increased urea production, decreased urea elimination, or a combination of the two.
By far, the highest serum BUN levels occur when the kidneys are not functioning correctly and cannot eliminate urea effectively relative to the demand. The leading cause is increased protein catabolism and consequent increased urea production due to excess consumption of animal protein.
Animal proteins such as dairy products, poultry, eggs, and red meat require more digestive juices, water, and healthy gut bacteria to be processed than versus plant-based fats and proteins. Adding just 10 percent saturated fat (primarily from animal sources) increases the rate at which stomach acid converts proteins into nitrosamines and decreases the production of nitric oxide, especially when these foods are cooked at high temperatures such as by grilling, frying, roasting, and microwaving.
Nitrosamines increase the amount of ammonia and urea the liver must produce and the kidneys must excrete in urine. Elevated urea levels in the blood are directly linked to higher conversion of nitrates into nitrosamines instead of nitric oxide, which increases the risk of developing chronic kidney disease, cardiovascular disease, cancer, and many other chronic diseases. On the other hand, diets high in unsaturated fats (primarily derived from plant sources such as olive oil, avocado oil, and coconut oil) improve nitric oxide production. Nitric oxide is critical for blood to flow, the brain to function, to make proteins and nucleic acids, such as DNA and RNA, and to prevent the overgrowth of cancer cells and pathogenic microorganisms.
Dehydration, insufficient production of hydrochloric acid and digestive enzymes in the stomach, and dysbiosis (deficient healthy bacteria in the gut relative to pathogenic bacteria) – all required for breaking down protein and other macromolecules – overwork the gut and increase the liver’s conversion of toxic protein byproducts into the less toxic urea. The kidneys must work harder to excrete urea in the urine and conserve water. The consequent increase in water conservation by the kidneys and increased blood urea levels leads to accelerated dehydration and cell death.
Suppose the root cause is not adequately addressed. In that case, the kidneys struggle to balance electrolytes, blood and urine acidity, alkalinity and fluid levels, and blood pressure and make mature red blood cells. Other organ functions are also negatively impacted. For example, the liver struggles with detoxifying the blood, balancing hormone levels, cholesterol metabolism, and many other essential functions. The digestive system struggles to break down food, absorb essential nutrients, and produce nitric oxide, which is critical for making energy and for every organ in the body to function correctly. This inevitably leads to poorly digested cholesterol depositing on the inside lining of blood vessels, triggering an inflammatory response and restricting blood flow throughout the body.
The other leading causes of high BUN levels are increased tissue damage, e.g., trauma, major surgery, gastrointestinal bleeding[13], starvation, severe infection, and certain drugs. They all induce a catabolic state with increased protein breakdown and consequent increase in urea production, its reabsorption by the kidneys, and increased blood urea levels. Elevated blood urea levels damage cell walls, inhibit cell division, increase gene mutation, interfere with gene expression, and increase inflammation. Pro-inflammatory cytokines, IL-1, TNF-α, IFN-γ, and IL-6, for example, can interfere with many hormones [SCF (stem cell factor), IL-3, GM-SCF (granulocyte-macrophage stem cell factor), erythropoietin, androgens, and thyroxine] – critical for RBC formation in the bone marrow and kidneys[18]. It can also interfere with the enzyme methylenetetrahydrofolate reductase (MTHFR)’s ability to activate folate (Vitamin B9)[19].
Low circulatory states such as heart failure [14], dehydration [15], substantial blood loss, and hypovolemic shock where the kidneys are not adequately perfused all invoke an adaptive response associated with increased plasma/serum urea. The plasma creatinine and GFR may remain the same temporarily.
The most widely cited drug group for elevated BUN levels are corticosteroids and other synthetic hormones commonly prescribed in conventional medicine.
Low BUN Levels (i.e. urea <1.46 mmol/L or BUN <4.0 mg/dL)
Since urea concentration in plasma or serum reflects the balance between urea production and urea elimination in urine, reduced plasma/serum urea can be caused by decreased urea production, increased urinary urea excretion, or a combination of the two.
Two leading physiologic causes of reduced BUN concentration are a low-protein diet and pregnancy.
A low-protein diet is associated with reduced urea production and a consequent tendency to reduce plasma/serum urea concentration.
The reduced plasma/serum urea that commonly occurs during pregnancy is due to the combined effect of reduced urea production and increased urea excretion [16].
Pathological causes of reduced urea concentration are largely confined to malnutrition due to a low whole-food plant-based diet rich in dark leafy vegetables or long-standing food intolerance to animal proteins and GMO grains such as wheat, corn, and soy. It leads to decreased hydrochloric acid production in the stomach and a low concentration of healthy bacteria relative to pathogenic bacteria. Over time, this inhibits the metabolism of amino acids by the liver and interferes with the urea cycle.
Inherited deficiency of any one of the five enzymes of the urea cycle describes a rare group of conditions (called the urea cycle defects) that can give rise to reduced urea synthesis and consequent reduced plasma/serum urea concentration.[17]
Overhydration caused by an inability to absorb water effectively overworks the kidneys, increases urea excretion in the urine, and decreases plasma/serum urea.
Summary
Urea is a waste product of protein metabolism that is excreted by the kidneys in urine. Kidney and liver disease is associated with reduced urea excretion and consequent rise in blood urea concentration.
The most common causes of abnormal BUN or urea levels are dehydration, high animal protein and/or GMO grain diet, and inadequate consumption of whole foods from plants rich in dark leafy vegetables. Some medications, such as corticosteroids and other synthetic hormones, can also directly impact BUN levels.
Sources:
- Pagana KD, Pagana TJ, Pagana TN. Mosby’s Diagnostic and Laboratory Test Reference. 15th ed. St. Louis, MO: Elsevier; 2021.
- “Comparisons of hazard ratios when laboratory results were within the normal range demonstrated that blood albumin and blood urea nitrogen (BUN) were both negatively associated with mortality and alkaline phosphatase (AP) was positively associated with mortality.” Hu G, Duncan AW. Associations between selected laboratory tests and all-cause mortality. J Insur Med. 2013;43(4):208-220. [PubMed]
- Increased discharge serum urea (> 16 mg/dL) was a predictor of increased mortality in heart failure patients. Gotsman I, Zwas D, Planer D, Admon D, Lotan C, Keren A. The significance of serum urea and renal function in patients with heart failure. Medicine (Baltimore). 2010;89(4):197-203. [PubMed]
- A steady increase in mortality was predicted for Medicare patients (aged > or = 65 years) who were hospitalized for myocardial infarction (n = 44,437) who had increased levels of BUN > 17 mg/dL(> 6.1 mmol/L), creatinine > 1.0 mg/dL (> 88.4 micromol/L), and eGFR < 100 mL/min per 1.73 m2 and in patients who experienced heart failure who had increased levels of BUN > 16 mg/dL (> 5.7 mmol/L), creatinine > 1.1 mg/dL (> 97.2 micromol/L), and a Mayo eGFR <= 90 mL/min per 1.73 m2. Smith GL, Shlipak MG, Havranek EP, et al. Serum urea nitrogen, creatinine, and estimators of renal function: mortality in older patients with cardiovascular disease. Arch Intern Med. 2006;166(10):1134-1142. [PubMed]
- A BUN ≥ 30 mg/dL was associated with a nearly 2-fold increased risk of mortality…” Sullivan DH, Sullivan SC, Bopp MM, Roberson PK, Lensing SY. BUN as an Independent Predictor of Post-Hospital-Discharge Mortality among Older Veterans. J Nutr Health Aging. 2018;22(7):759-765. [PubMed]
- https://www.earth.com/earthpedia-articles/why-is-the-nitrogen-cycle-so-important/
- https://acutecaretesting.org/en/articles/urea-and-the-clinical-value-of-measuring-blood-urea-concentration
- Watford M. The urea cycle: Teaching intermediary metabolism in a physiological setting. Biochem Mol Biol Educ 2003; 31, 5: 289-97.
- Lamb E et tal. Kidney Function Tests (Chapter 25) In: Tietz Textbook of Clinical Chemistry and Molecular Diagnostics. By: Burtis CA, Ashwood ER, Bruns DE. Elsevier Health Sciences; 2012. Available from: URL:https://books.google.dk/books?id=BBLRUI4aHhkC.
- Bowker LK et tal. Raised blood urea in the elderly: a clinical and pathological study. Postgrad Med J 1992; 68, 797: 174-79.
- Chung J et tal. P15 Elevated urea in the elderly: Age or EGFR – a database analysis. [cited 2015 Nov 26]. Available from:www.aacb.asn.au/documents/item/960.
- Musch W et tal. Age-related increase in plasma urea level and decrease in fractional urea excretion: clinical application in the syndrome of inappropriate secretion of antidiuretic hormone. CJASN 2006; 1, 5: 909-14.
- Witting MD et tal. ED predictors of upper gastrointestinal tract bleeding in patients without hematemesis. Am J Emerg Med 2006; 24, 3: 280-85.
- Aronson D et tal. Elevated blood urea nitrogen level as a predictor of mortality in patients admitted for decompensated heart failure. The Am J Med 2004; 116, 7: 466-73.
- Mehta AR. Why does the plasma urea concentration increase in acute dehydration? Advances in physiology education 2008; 32, 4: 336.
- Kalhan SC. Protein metabolism in pregnancy. Am J Clin Nutr 2000; 71, 5 Suppl: 1249S-55S.
- Salek J et tal. Recurrent liver failure in a 25-year-old female. Liver transplantation: official publication of the American Association for the Study of Liver
Diseases and the International Liver Transplantation Society 2010; 16, 9: 1049-53. - https://www.ncbi.nlm.nih.gov/pmc/articles/PMC4651743/
- Gao, X., Lee, H. Y., Li, W., Platt, R. J., Barrasa, M. I., Ma, Q., … & Lodish, H. F. (2017). Thyroid hormone receptor beta and NCOA4 regulate terminal erythrocyte differentiation. Proceedings of the National Academy of Sciences, 114(38), 10107-10112.
- ROSE WC. The amino acid requirements of adult man. Nutr Abstr Rev. 1957 Jul;27(3):631-47.
- Benjamin O, Lappin SL. StatPearls. StatPearls Publishing; Treasure Island (FL): Jul 19, 2022. Kwashiorkor.